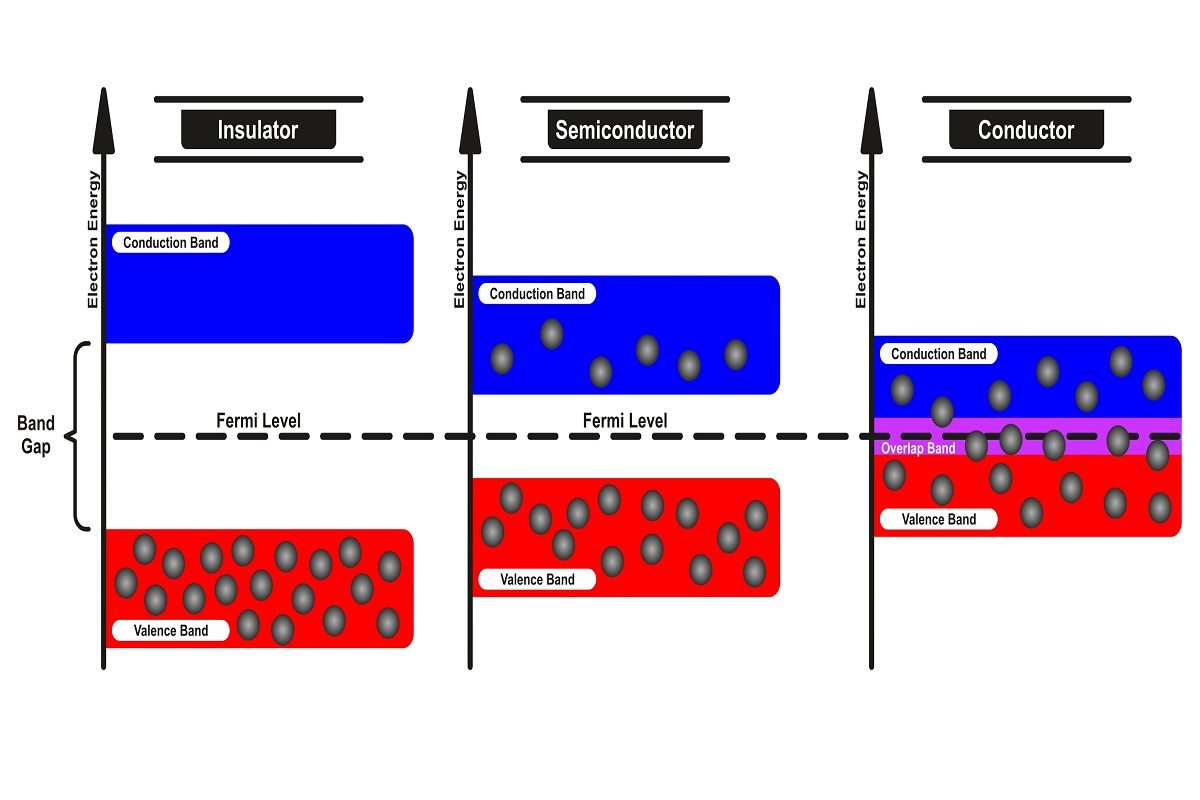
The concept of Fermi level is central to our understanding of the electronic structure of materials. Named after the renowned physicist Enrico Fermi, the Fermi level represents the energy level at which a material’s electron distribution is in equilibrium at absolute zero temperature. It serves as a reference point for understanding various phenomena in solid-state physics and plays a vital role in determining the electrical and thermal properties of materials.
In this article, we will explore 16 unbelievable facts about the Fermi level that will deepen your understanding of its significance in the world of chemistry and materials science. From its influence on conductivity and energy band diagrams to its relationship with doping and Fermi-Dirac statistics, we will delve into the intriguing aspects of the Fermi level that make it such a fascinating concept in the field of solid-state physics.
Key Takeaways:
- The Fermi level determines how well a material conducts electricity, and it can be influenced by temperature and impurities, affecting everything from electronic devices to chemical reactions.
- Understanding the Fermi level is like having a secret key to unlock new material properties and create amazing technologies that can change the world!
The Fermi level is a crucial concept in solid-state physics.
The Fermi level, named after the Nobel laureate Enrico Fermi, represents the highest energy state that an electron can occupy at absolute zero temperature in a solid. It plays a vital role in determining the electrical properties of materials.
The Fermi level determines the conductivity of a material.
The position of the Fermi level within the energy band structure of a material determines whether it behaves as an insulator, semiconductor, or conductor. In insulators, the Fermi level lies within the energy band gap, making it impossible for electrons to move freely. In semiconductors, the Fermi level lies close to the energy band edge, allowing limited electron flow. In conductors, the Fermi level lies within the energy band, enabling electrons to move freely and conduct electricity.
The Fermi level is temperature-dependent.
As temperature increases, the distribution of electrons across energy levels changes, causing the Fermi level to shift. This temperature dependence of the Fermi level affects the electrical conductivity of materials. In metals, for example, increasing temperature can lead to an increase in electron scattering, resulting in higher resistivity.
The Fermi level can be influenced by doping.
Doping is the process of intentionally introducing impurities into a material to alter its electrical properties. By introducing impurities with different energy levels, such as donor or acceptor atoms, the position of the Fermi level can be modified. This manipulation allows for the control of conductivity and the creation of p-type or n-type semiconductors.
The Fermi level affects electronic device performance.
In electronic devices, the position of the Fermi level determines the energy barrier required for electron flow across different materials, such as in diodes or transistors. By optimizing the Fermi level alignment, device performance, efficiency, and speed can be improved.
The Fermi level plays a role in quantum mechanics.
In quantum mechanics, the Fermi level determines the probability of finding an electron in a particular energy state. The Fermi-Dirac distribution function describes the occupation of electron energy states at different temperatures. Understanding the Fermi level is essential in studying phenomena such as superconductivity, quantum tunneling, and electron transport in nanoscale devices.
The Fermi level can be measured experimentally.
Various experimental techniques, such as photoemission spectroscopy and Hall effect measurements, can be used to determine the position of the Fermi level in materials. These measurements provide valuable insights into the electronic structure and conductivity of solids.
The Fermi level affects chemical reactions.
The position of the Fermi level can influence the energy required for electron transfer during chemical reactions. In catalysis, for example, the alignment of the Fermi level with the energy levels of reactants can enhance or inhibit the reaction rate. Understanding the Fermi level is crucial in designing efficient catalysts for various chemical processes.
The Fermi level is sensitive to external factors.
External factors such as doping, strain, and electric fields can modify the position of the Fermi level. This sensitivity allows for the tuning and control of the electronic properties of materials, opening up possibilities for advanced device engineering and nanotechnology.
The Fermi level affects the thermoelectric properties of materials.
The Fermi level plays a critical role in determining the thermoelectric efficiency of materials, which relates to their ability to convert heat into electrical energy. Optimizing the Fermi level position can enhance the thermoelectric performance, enabling more efficient energy harvesting and waste heat recovery.
The Fermi level is essential in understanding band theory.
Band theory describes the behavior of electrons in solids and is based on the concept of the Fermi level. Understanding the Fermi level is crucial in interpreting energy band diagrams, band gaps, and the electronic properties of materials.
The Fermi level can exhibit quantization effects.
In low-dimensional systems, such as quantum wells, wires, and dots, the Fermi level can display quantization effects due to the confinement of electrons along specific directions. These effects lead to discrete energy levels and have significant implications for electronic and optical devices operating at the nanoscale.
The Fermi level affects the optical properties of materials.
The position of the Fermi level influences the absorption and emission of light in materials. In semiconductors, for example, the energy difference between the Fermi level and the valence or conduction band edge determines the optical properties, such as the color of emitted light in light-emitting diodes.
The Fermi level can undergo shifts under external pressure.
Applying external pressure to materials can cause the Fermi level to shift. This pressure-induced shift can result in changes in electronic and structural properties, influencing phenomena such as metal-insulator transitions and phase transitions.
The Fermi level is important in the study of energy levels and electron transport.
The Fermi level provides a reference energy that helps in understanding phenomena related to energy levels, electron transport, and charge carriers in materials. It is a fundamental concept in solid-state physics and is widely used in the analysis and design of electronic devices and materials.
The Fermi level holds the key to unlocking new material properties and applications.
Developing a deep understanding of the Fermi level and its implications in various materials paves the way for the discovery and design of novel materials with tailored electronic properties. This knowledge can lead to advancements in fields such as energy conversion, semiconductor technology, and quantum computing.
Conclusion
The Fermi level is a crucial concept in understanding the behavior of electrons in materials. From its origins in quantum mechanics to its applications in semiconductors and superconductors, the Fermi level has a profound impact on the electronic properties of materials.In this article, we have explored 16 unbelievable facts about the Fermi level. We have learned that the Fermi level represents the energy at which electrons in a solid are distributed, and it determines whether a material is a conductor, insulator, or semiconductor. We have also discovered that the Fermi level plays a key role in phenomena such as bandgap, doping, and charge carrier concentration.Furthermore, we have delved into the fascinating aspects of the Fermi level, such as its relationship with temperature, its effect on electrical conductivity, and its connection to thermoelectric properties. We have also explored the impact of impurities, defects, and magnetic fields on the Fermi level.Understanding the Fermi level opens up a world of possibilities in materials science and electronics. From designing more efficient solar cells to developing faster computer chips, the Fermi level continues to be a subject of great interest and ongoing research.In conclusion, the Fermi level is a fundamental concept that underlies the behavior of electrons in materials. Its exploration leads to amazing discoveries and enables advancements in various technological fields.
FAQs
1. What is the Fermi level?
The Fermi level is the energy level at which the probability of an electron being occupied is 0.5. It represents the highest occupied energy level at absolute zero temperature.
2. How does the Fermi level affect electrical conductivity?
The Fermi level determines whether a material is a conductor, insulator, or semiconductor. In conductors, the Fermi level lies within the conduction band, allowing for the easy flow of electrons. In insulators, the Fermi level lies within the bandgap, creating a large energy barrier for electron movement. In semiconductors, the Fermi level lies near the middle of the bandgap, allowing for some conductivity through the presence of thermally excited electrons.
3. Does the Fermi level change with temperature?
Yes, the Fermi level shifts with temperature. As the temperature increases, the energy distribution of electrons changes, causing the Fermi level to shift towards higher energies in metals and doped semiconductors.
4. How does doping affect the Fermi level?
Doping introduces impurities into a material, thereby altering its electronic properties. In n-type doping, additional electrons are added, causing the Fermi level to move closer to the conduction band. In p-type doping, electron deficiencies are created, causing the Fermi level to move closer to the valence band.
5. Can magnetic fields affect the Fermi level?
Yes, magnetic fields can influence the behavior of electrons and, consequently, the Fermi level. In the presence of a magnetic field, the energy levels split into separate bands, referred to as Landau levels. The Fermi level then occupies the highest filled Landau level.
Was this page helpful?
Our commitment to delivering trustworthy and engaging content is at the heart of what we do. Each fact on our site is contributed by real users like you, bringing a wealth of diverse insights and information. To ensure the highest standards of accuracy and reliability, our dedicated editors meticulously review each submission. This process guarantees that the facts we share are not only fascinating but also credible. Trust in our commitment to quality and authenticity as you explore and learn with us.