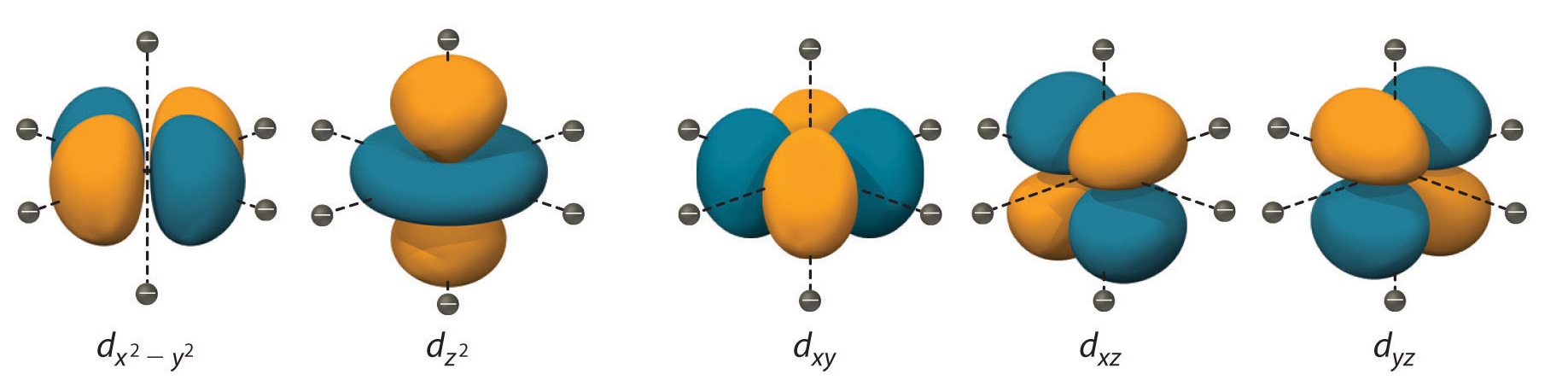
Crystal Field Theory is a fundamental concept in the field of chemistry that provides invaluable insights into the behavior and properties of transition metal complexes. By examining the interaction between metal ions and their surrounding ligands, this theory allows us to understand the magnetic, optical, and catalytic properties of these complexes, and how they influence the overall reactivity of the system.
In this article, we will delve into the intriguing world of Crystal Field Theory and explore 20 mind-blowing facts that will deepen your understanding of this powerful tool. From the origins of the theory to its applications in various branches of chemistry, get ready to be amazed by the fascinating realm of Crystal Field Theory. So buckle up and let’s explore the secrets hidden within the crystal lattice!
Key Takeaways:
- Crystal Field Theory explains why transition metal compounds have different colors and magnetic properties, helping scientists predict and understand their behavior.
- By considering the arrangement of ligands and d orbitals, Crystal Field Theory can be used to predict the absorption spectrum and stability of transition metal complexes, with practical applications in various industries.
Crystal Field Theory explains the colors of transition metal compounds.
Crystal Field Theory (CFT) is a fundamental concept in inorganic chemistry that provides insight into the colors observed in transition metal compounds. It explains how the arrangement of ligands around a metal ion influences the energy levels of the d orbitals and leads to the absorption and emission of specific wavelengths of light.
CFT was developed by physicists Hans Bethe and John Hasbrouck van Vleck.
In the 1930s, Bethe and Van Vleck independently formulated Crystal Field Theory as a model to describe the behavior of transition metal complexes. Their groundbreaking work revolutionized the understanding of the electronic structure of these compounds.
The ligands in CFT are considered point charges.
In Crystal Field Theory, ligands surrounding the metal ion are treated as point charges. The negatively charged ligands influence the energy levels of the metal’s d orbitals, causing them to split into higher energy and lower energy sets.
CFT explains the difference between high spin and low spin complexes.
Crystal Field Theory provides an explanation for the distinction between high spin and low spin complexes. In high spin complexes, the d orbitals are not completely filled, resulting in higher energy levels. In low spin complexes, the d orbitals are more fully filled, leading to lower energy levels.
CFT helps predict the magnetic properties of transition metal complexes.
By considering the arrangement and occupancy of the d orbitals in transition metal complexes, Crystal Field Theory can predict whether a complex will be paramagnetic or diamagnetic. Paramagnetic complexes have unpaired electrons and are attracted to a magnetic field, while diamagnetic complexes have only paired electrons and are not affected by a magnetic field.
The splitting of d orbitals in CFT is known as crystal field splitting.
Crystal field splitting refers to the energy difference between the higher energy set and the lower energy set of d orbitals in Crystal Field Theory. This splitting determines the color observed in transition metal compounds when they absorb or emit light.
CFT can explain the stability of octahedral and tetrahedral complexes.
Crystal Field Theory provides a rationale for the preference of transition metal ions to form octahedral or tetrahedral complexes. Octahedral complexes have a greater energy difference between the higher and lower energy sets of d orbitals, making them more stable than tetrahedral complexes.
CFT can explain the phenomenon of charge transfer transitions.
One of the key features of Crystal Field Theory is its ability to explain charge transfer transitions in transition metal complexes. These transitions involve the transfer of an electron from a ligand orbital to a metal orbital, resulting in the absorption or emission of light of a specific wavelength.
The strength of the crystal field depends on the nature of the ligands.
The strength of the crystal field experienced by a transition metal ion is influenced by the nature of the ligands surrounding it. Ligands with a high charge and small size result in a stronger crystal field, leading to larger energy differences between the d orbitals.
CFT can account for the different oxidation states of transition metal ions.
Crystal Field Theory offers an explanation for the various oxidation states exhibited by transition metal ions. The arrangement and occupancy of the d orbitals are affected by the oxidation state, influencing the electronic structure and behavior of the metal complex.
The use of spectroscopic techniques can provide experimental evidence for CFT.
Crystal Field Theory makes predictions about the colors and electronic transitions of transition metal complexes. Spectroscopic techniques such as UV-Vis spectroscopy and electronic absorption spectroscopy can be used to confirm and study these predictions, providing experimental evidence for CFT.
CFT is a simplified model and does not account for all aspects of transition metal complexes.
Although Crystal Field Theory has proven to be a valuable tool in understanding the electronic structure of transition metal complexes, it is a simplified model that neglects certain factors such as covalency and orbital overlap. More advanced theories, such as Ligand Field Theory and Molecular Orbital Theory, provide a more comprehensive understanding of these systems.
The coordination number affects the crystal field splitting energy.
The number of ligands surrounding a transition metal ion, known as the coordination number, influences the crystal field splitting energy. Higher coordination numbers generally result in larger splitting energy, leading to a more pronounced color in the compound.
CFT can explain the phenomenon of Jahn-Teller distortion.
Jahn-Teller distortion is a phenomenon observed in transition metal complexes where the complex undergoes a geometric distortion to maximize its stability. Crystal Field Theory can provide insights into this distortion by considering the energy levels and occupancy of the d orbitals.
CFT can be used to predict the absorption spectrum of transition metal complexes.
By analyzing the crystal field splitting energy and the nature of ligands, Crystal Field Theory can predict the wavelengths at which transition metal complexes will absorb light. This information is useful in fields such as materials science and spectroscopy.
CFT is applicable to a wide range of transition metal elements.
Crystal Field Theory is not limited to specific transition metal elements and can be applied to a wide range of them. The principles and concepts of CFT remain consistent across different transition metals, allowing for a comprehensive understanding of their behavior.
CFT can explain the effect of ligand field strength on the stability of complexes.
The strength of the ligand field, which is determined by the ligands surrounding the transition metal ion, can influence the stability of the complex. Stronger ligand fields result in higher crystal field splitting energies, leading to more stable complexes.
The crystal field splitting energy can be determined using spectroscopic techniques.
Spectroscopic techniques such as electronic absorption spectroscopy can be used to determine the crystal field splitting energy of transition metal complexes. This information provides insight into the electronic structure and bonding properties of these compounds.
CFT is commonly used in the field of coordination chemistry.
Crystal Field Theory is widely employed in the field of coordination chemistry to understand and predict the behavior of transition metal complexes. It serves as a foundational theory that forms the basis for more advanced theories and models.
CFT has practical applications in various industries.
Crystal Field Theory has practical applications in industries such as materials science, pharmaceuticals, and catalysis. By understanding the electronic properties and behavior of transition metal complexes, scientists and engineers can design and optimize materials and processes for specific purposes.
Conclusion
Crystal Field Theory is a fascinating concept that has revolutionized our understanding of the behavior of transition metal complexes. By considering the effects of crystal field splitting on the electrons present in the d-orbitals of the metal ion, we can explain and predict various properties and phenomena, such as color, magnetic behavior, and spectroscopic features.
Throughout this article, we have uncovered 20 remarkable facts about Crystal Field Theory. We have explored the concept of ligand-field splitting, the different types of crystal field environments, and the significance of ligand coordination on the electronic structure of transition metal complexes. We have also examined the spectrochemical series, the use of Crystal Field Theory in explaining geometric isomerism, and its applications in various fields including biology, medicine, and materials science.
By delving into the intricacies of Crystal Field Theory, we gain a deeper understanding of the behavior of transition metal complexes and their relevance in numerous practical applications. Whether you are a student of chemistry, a researcher, or simply curious about the fascinating world of materials science, Crystal Field Theory is undoubtedly an essential concept to explore.
FAQs
Q: What is Crystal Field Theory?
A: Crystal Field Theory is a model used in chemistry to explain the electronic structure and properties of transition metal complexes. It considers the effects of the electric field generated by ligands surrounding a transition metal ion on the energies of the d-orbitals.
Q: How does Crystal Field Theory explain the color of transition metal complexes?
A: Crystal Field Theory explains the color of transition metal complexes by considering the absorption of certain wavelengths of light. When ligands generate a crystal field, they cause the d-orbitals of the metal ion to split into different energy levels. Electrons in the lower energy levels absorb specific wavelengths of light, resulting in the complementary color being observed.
Q: What are the different types of crystal field environments?
A: The two main types of crystal field environments are octahedral and tetrahedral. In an octahedral environment, the metal ion is surrounded by six ligands arranged in an octahedral shape. In a tetrahedral environment, the metal ion is surrounded by four ligands arranged in a tetrahedral shape.
Q: How is Crystal Field Theory applied in biology and medicine?
A: Crystal Field Theory is utilized in understanding the behavior of metal ions in biological systems, such as enzymes and proteins. It helps in investigating the role of transition metal ions in catalytic reactions and their interactions with ligands. Crystal Field Theory is also employed in the development of metal-based drugs for various medical applications.
Q: Can Crystal Field Theory explain geometric isomerism?
A: Yes, Crystal Field Theory can explain geometric isomerism. It considers the different ligand-field splittings that lead to distinct energy levels for the d-orbitals, resulting in different geometric isomers. This theory helps in understanding the preference of transition metal complexes for certain spatial arrangements.
Was this page helpful?
Our commitment to delivering trustworthy and engaging content is at the heart of what we do. Each fact on our site is contributed by real users like you, bringing a wealth of diverse insights and information. To ensure the highest standards of accuracy and reliability, our dedicated editors meticulously review each submission. This process guarantees that the facts we share are not only fascinating but also credible. Trust in our commitment to quality and authenticity as you explore and learn with us.