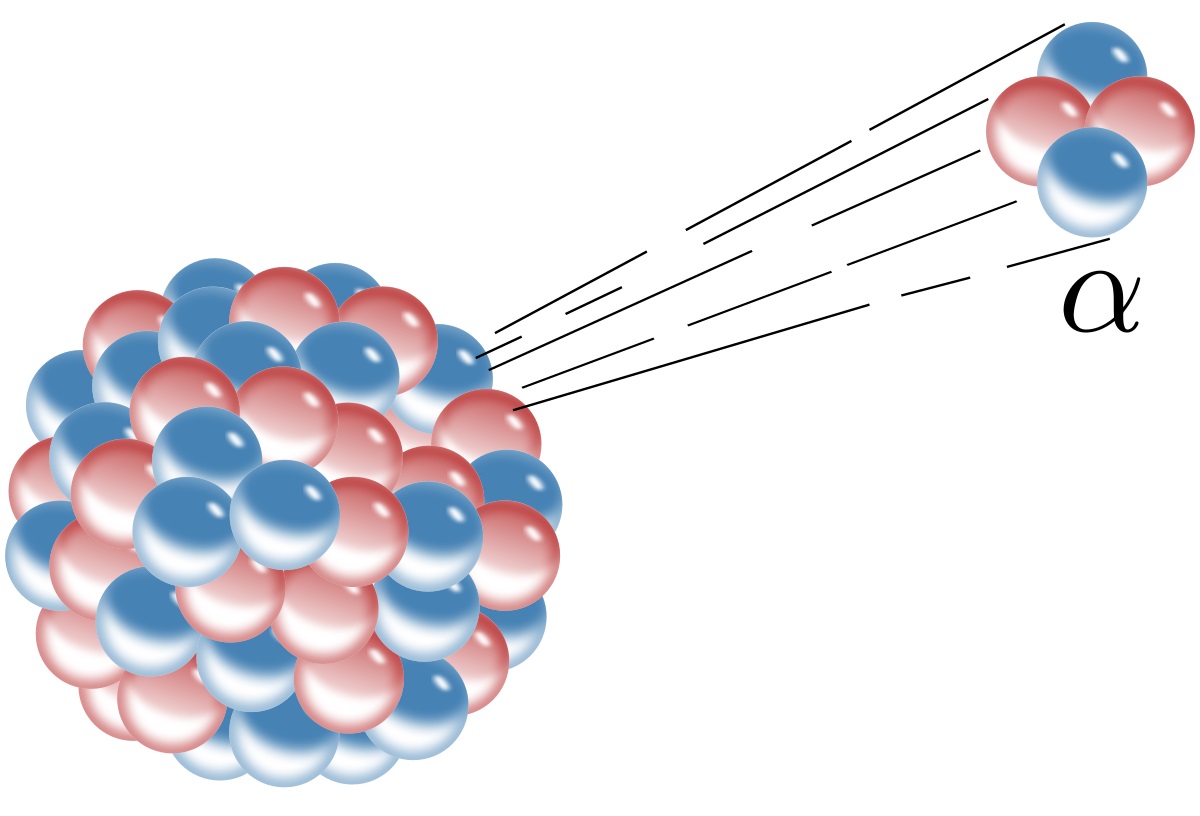
Sure, here's the introduction wrapped in
tags:
Radioactive decay is a fascinating and fundamental process that plays a crucial role in various scientific fields, including chemistry, physics, and geology. Understanding the intricacies of radioactive decay is not only essential for academic purposes but also has practical applications in medicine, energy production, and environmental monitoring. In this article, we will delve into 18 intriguing facts about radioactive decay, shedding light on its mechanisms, significance, and real-world implications. From the discovery of radioactivity to the different types of radioactive decay and its impact on everyday life, this comprehensive exploration will unveil the captivating world of nuclear transformations and their enduring impact on the world around us.
Key Takeaways:
- Radioactive decay is a natural process that occurs in certain elements, leading to the release of radiation and the formation of different elements. It has practical applications in fields like medicine and energy production.
- Understanding radioactive decay is crucial for determining the age of materials and has implications for space exploration. It also plays a key role in driving geological processes and sustaining the Earth’s internal dynamics.
Radioactive decay is a natural process.
Radioactive decay is a spontaneous process in which an unstable atomic nucleus loses energy by emitting radiation. This process is entirely random and cannot be influenced by any external factors.
It occurs in isotopes of elements.
Isotopes are variants of a particular chemical element, which have the same number of protons but different numbers of neutrons. Radioactive decay occurs in certain isotopes of elements, leading to the formation of different elements and the release of radiation.
There are different types of radioactive decay.
The three primary types of radioactive decay are alpha decay, beta decay, and gamma decay. Each type involves the release of different particles and energy, resulting in the transformation of the original nucleus.
It is measured using half-life.
Half-life is the time required for half of the radioactive nuclei in a sample to undergo decay. It is a crucial measure in understanding the stability and decay rate of a radioactive substance.
Radioactive decay plays a vital role in radiometric dating.
By analyzing the decay of radioactive isotopes in geological and archaeological samples, scientists can determine the age of materials, such as rocks and fossils, through a process known as radiometric dating.
It has numerous practical applications.
Radioactive decay is utilized in various fields, including medicine, energy production, and environmental monitoring. For instance, it is employed in medical imaging, power generation in nuclear reactors, and detecting environmental pollution.
Some elements have no stable isotopes.
Several elements, such as technetium and promethium, do not have any stable isotopes and undergo radioactive decay. These elements are exclusively produced through artificial processes or occur as by-products of nuclear reactions.
It is a fundamental concept in nuclear physics.
Understanding radioactive decay is crucial in nuclear physics, as it governs the behavior of atomic nuclei and the release of energy in nuclear reactions. This knowledge forms the basis of nuclear power and weaponry.
Radioactive decay can be harmful to living organisms.
Exposure to high levels of radioactive decay products can be detrimental to living organisms, causing genetic mutations, radiation sickness, and an increased risk of cancer. Proper safety measures are essential when working with radioactive materials.
It is a source of background radiation.
Natural background radiation, which includes the effects of radioactive decay from sources like radon gas and cosmic rays, is constantly present in the environment. This radiation contributes to overall exposure levels for humans.
Radioactive decay can be shielded.
Various materials, such as lead and concrete, are effective in shielding against the radiation emitted during radioactive decay. This principle is applied in the design of protective barriers for nuclear facilities and equipment.
It is a continuous process.
Radioactive decay occurs at a constant and predictable rate, allowing scientists to study the behavior of radioactive isotopes over time. This process is unaffected by changes in temperature, pressure, or chemical reactions.
It is a form of nuclear transmutation.
During radioactive decay, the original nucleus undergoes a transmutation, resulting in the formation of a different element. This transformation is a fundamental concept in nuclear chemistry and particle physics.
Radioactive decay can be induced artificially.
Artificially induced radioactive decay is utilized in various applications, including medical treatments and scientific research. By bombarding stable nuclei with particles, scientists can create radioactive isotopes for specific purposes.
It is a source of geothermal heat.
The decay of radioactive isotopes within the Earth’s crust contributes to geothermal heat production. This natural process is harnessed in geothermal energy systems for heating and electricity generation.
Radioactive decay follows exponential decay kinetics.
The rate of radioactive decay follows an exponential decay curve, where the amount of radioactive material decreases rapidly at first and then gradually approaches stability. This behavior is described by mathematical equations and models.
It is a key factor in the formation of Earth’s core.
The heat generated from radioactive decay in the Earth’s core plays a significant role in driving geological processes, including mantle convection and the generation of the planet’s magnetic field. This heat sustains the Earth’s internal dynamics.
Radioactive decay has implications for space exploration.
Understanding the behavior of radioactive isotopes and their decay rates is essential for space missions involving long-duration space travel and the exploration of extraterrestrial environments. Radioactive power sources are utilized in certain spacecraft and rovers for extended missions.
Conclusion
Understanding the process of radioactive decay is crucial for various scientific fields and everyday life. The 18 facts about radioactive decay shed light on its significance, applications, and potential risks. From the fundamental concept of decay to its role in dating techniques and energy production, the intricate nature of this phenomenon is both fascinating and essential. As we continue to delve deeper into the properties of radioactive elements, we uncover new opportunities for medical advancements, environmental monitoring, and energy innovations. By comprehending these facts, we can appreciate the intricate balance between the benefits and hazards associated with radioactive decay, paving the way for responsible utilization and continued scientific exploration.
FAQs
What is radioactive decay?
Radioactive decay is the process by which an unstable atomic nucleus loses energy by emitting radiation, ultimately transforming into a more stable nucleus.
What are some practical applications of radioactive decay?
Radioactive decay is utilized in various fields, including medical imaging, carbon dating, and nuclear power generation.
How does radioactive decay contribute to nuclear energy production?
Radioactive decay generates heat, which is harnessed to produce steam and drive turbines, ultimately generating electricity in nuclear power plants.
What safety measures are in place to mitigate the risks associated with radioactive decay?
Strict regulations, shielding materials, and proper waste management are implemented to ensure the safe handling and disposal of radioactive materials.
Can radioactive decay be reversed?
No, radioactive decay is a spontaneous and irreversible process that occurs at a constant rate for each radioactive isotope.
How does radioactive decay contribute to medical treatments?
Radioactive isotopes are used in cancer treatments and diagnostic imaging, providing valuable tools for medical professionals.
What are the environmental implications of radioactive decay?
Proper monitoring and management of radioactive waste are essential to prevent environmental contamination and safeguard ecosystems.
How is the rate of radioactive decay measured?
The rate of decay is quantified using the concept of half-life, which represents the time required for half of the radioactive atoms in a sample to decay.
What are some common radioactive isotopes used in research and applications?
Isotopes such as carbon-14, uranium-235, and iodine-131 are frequently employed in scientific research, medical procedures, and industrial processes.
What are the key differences between alpha, beta, and gamma decay?
Alpha decay involves the emission of alpha particles, beta decay results in the release of beta particles, and gamma decay emits gamma rays, each with distinct properties and effects.
Radioactive decay's mysteries run deep, but your curiosity doesn't have to stop here. Explore nuclear decay's captivating facts, alpha particles' surprising traits, and beta particles' extraordinary characteristics. These related topics offer even more fascinating insights into the world of radioactivity and its role in shaping our universe. So, keep reading, keep learning, and prepare to be amazed by the incredible science behind these fundamental processes.
Was this page helpful?
Our commitment to delivering trustworthy and engaging content is at the heart of what we do. Each fact on our site is contributed by real users like you, bringing a wealth of diverse insights and information. To ensure the highest standards of accuracy and reliability, our dedicated editors meticulously review each submission. This process guarantees that the facts we share are not only fascinating but also credible. Trust in our commitment to quality and authenticity as you explore and learn with us.