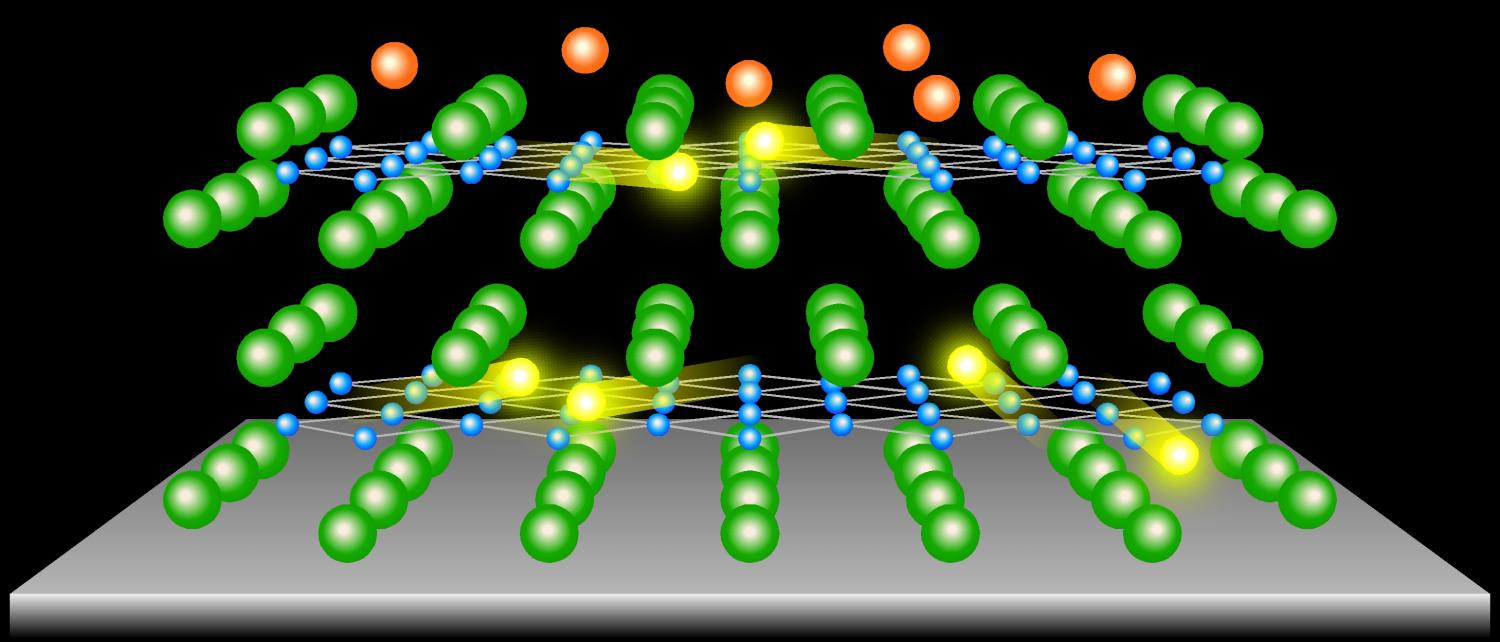
High-temperature superconductivity is a fascinating and complex field of study within the realm of physics and materials science. Superconductivity refers to the phenomenon where a material exhibits zero electrical resistance, allowing for the efficient flow of electric current without any energy losses. What makes high-temperature superconductivity so intriguing is that it occurs at temperatures above the boiling point of liquid nitrogen, which is relatively high compared to conventional superconductors.
In this article, we will delve into the astonishing facts surrounding high-temperature superconductivity. From its discovery to the potential applications and the challenges faced by scientists, we will explore the groundbreaking advancements in this field that have the potential to revolutionize various industries. So, brace yourself for an enlightening journey into the world of high-temperature superconductivity, where seemingly impossible feats become a reality.
Key Takeaways:
- High-temperature superconductors can conduct electricity without any resistance, which could revolutionize power transmission and lead to energy-efficient electrical devices.
- The discovery of high-temperature superconductivity has opened up new possibilities in fields like quantum computing, particle accelerators, and renewable energy storage, sparking interdisciplinary collaborations and ongoing research.
High-temperature superconductors can conduct electricity with zero resistance.
High-temperature superconductivity refers to the phenomenon where certain materials can conduct electricity without any resistance when cooled below a specific critical temperature.
The critical temperature for high-temperature superconductivity is higher than that of conventional superconductors.
Unlike conventional superconductors, which require extremely low temperatures close to absolute zero, high-temperature superconductors can achieve superconductivity at comparatively higher temperatures.
High-temperature superconductors can be made from various types of compounds.
These compounds include cuprates, iron-based compounds, and fullerenes, among others. Each type offers unique properties and potential applications in different fields.
High-temperature superconductors have the potential to revolutionize power transmission.
The zero resistance property of high-temperature superconductors makes them highly efficient in carrying electric currents, resulting in reduced energy loss during transmission.
Scientists are still working to fully understand the mechanism behind high-temperature superconductivity.
Despite extensive research, the exact mechanism responsible for high-temperature superconductivity remains an ongoing scientific challenge.
High-temperature superconductors have the potential to enable levitating trains.
The zero resistance property and strong magnetic field interactions of high-temperature superconductors could lead to the development of superconducting magnetic levitation (Maglev) trains.
High-temperature superconductors can be used in sensitive scientific instruments.
Superconducting materials are employed in devices like magnetic resonance imaging (MRI) machines and particle accelerators due to their ability to generate powerful magnetic fields.
High-temperature superconductivity was discovered in 1986 by IBM researchers.
The breakthrough discovery of high-temperature superconductivity in copper-based materials was made by Karl Müller and Johannes Bednorz, earning them the Nobel Prize in Physics in 1987.
High-temperature superconductors have potential applications in quantum computing.
The quantum properties of superconducting materials make them promising candidates for the development of qubits, the fundamental units of information storage in quantum computers.
High-temperature superconductors can exhibit exotic properties like pseudogap and charge-density waves.
These unusual phenomena observed in high-temperature superconductors have sparked intense scientific interest and are still being investigated to unlock their full potential.
The discovery of high-temperature superconductivity opened up new directions in condensed matter physics.
This remarkable discovery spurred an explosion of research in the field of superconductivity, leading to advancements in our understanding of materials and their unique properties.
High-temperature superconductivity can be affected by external factors like pressure and magnetic fields.
The critical temperature and critical current of high-temperature superconductors can be significantly altered by applying pressure or exposing them to strong magnetic fields.
High-temperature superconductors have been used to create powerful electromagnets.
Superconducting materials have been utilized to build magnets for applications such as magnetic resonance imaging (MRI) and large-scale scientific experiments.
The search for even higher critical temperatures in superconducting materials is an active area of research.
Scientists are constantly exploring new materials and experimental techniques in pursuit of superconductors that can operate at even higher temperatures, which would have far-reaching technological implications.
High-temperature superconductivity can enable energy-efficient electrical devices.
The low energy dissipation of superconducting materials could lead to the development of high-efficiency electrical devices and contribute to the reduction of power consumption.
High-temperature superconductivity can be observed in both two-dimensional and three-dimensional materials.
Superconducting behavior has been demonstrated in thin films and layered structures, as well as in bulk crystals, expanding the range of potential applications.
High-temperature superconductivity could enable the development of more advanced particle accelerators.
Accelerators using superconducting materials can achieve higher energies and intensities, allowing for the exploration of fundamental particles and phenomena in greater detail.
The study of high-temperature superconductivity has sparked interdisciplinary collaborations.
Researchers from various fields, including materials science, physics, and engineering, are working together to unravel the mysteries of high-temperature superconductivity and explore its practical applications.
High-temperature superconductivity has the potential to revolutionize the storage and transmission of renewable energy.
Superconducting materials could play a crucial role in enhancing the efficiency and stability of energy storage systems and enabling efficient long-distance transmission of renewable energy sources.
Conclusion
High-temperature superconductivity is a fascinating and promising field of study that has the potential to revolutionize various technologies. From the discovery of unconventional superconductors to the exploration of their unique properties, scientists have made significant progress. The astonishing facts about high-temperature superconductivity highlight the immense potential of these materials, such as their ability to conduct electricity with zero resistance at higher temperatures.
With ongoing research and advancements, we can anticipate further breakthroughs in understanding and utilizing high-temperature superconductivity. These discoveries may lead to the development of more efficient power transmission systems, faster and more powerful computer processors, and even the creation of advanced magnetic levitation trains. The future of high-temperature superconductivity is bright and holds great promise for transforming numerous industries.
FAQs
1. What is high-temperature superconductivity?
High-temperature superconductivity refers to the phenomenon where certain materials can conduct electricity with zero electrical resistance at temperatures significantly higher than traditional superconductors.
2. How are high-temperature superconductors different from conventional superconductors?
Conventional superconductors require extremely low temperatures, typically close to absolute zero, to exhibit zero resistance. In contrast, high-temperature superconductors can maintain superconductivity at relatively higher temperatures, making them more practical for various applications.
3. How are high-temperature superconductors discovered?
High-temperature superconductors were discovered in the late 1980s through a serendipitous accident. Researchers stumbled upon a ceramic material that exhibited superconductivity at a temperature much higher than expected, marking the beginning of the exploration of high-temperature superconductivity.
4. What are some potential applications of high-temperature superconductors?
High-temperature superconductors have the potential to revolutionize power transmission, energy storage, medical imaging, and electronics. They can enable the development of more efficient and compact devices, such as faster computer processors and high-capacity batteries.
5. What challenges are involved in working with high-temperature superconductors?
One major challenge in working with high-temperature superconductors is the complex nature of these materials. Understanding and controlling their properties requires advanced techniques and knowledge. Additionally, the cost of producing high-temperature superconductors and integrating them into practical applications remains a hurdle.
6. Are there any current real-world applications of high-temperature superconductivity?
While high-temperature superconductivity is still in the research and development stage, there are some practical applications. These include high-field magnets used in research institutions, superconducting wires for power transmission, and magnetic levitation systems for transportation.
Superconductivity continues to amaze scientists and enthusiasts alike. Unraveling mysteries behind this phenomenon requires understanding superconductors and their unique properties. One crucial aspect is the critical temperature, which determines when materials transition into superconducting states. Exploring these concepts further can deepen our appreciation for this fascinating field.
Was this page helpful?
Our commitment to delivering trustworthy and engaging content is at the heart of what we do. Each fact on our site is contributed by real users like you, bringing a wealth of diverse insights and information. To ensure the highest standards of accuracy and reliability, our dedicated editors meticulously review each submission. This process guarantees that the facts we share are not only fascinating but also credible. Trust in our commitment to quality and authenticity as you explore and learn with us.