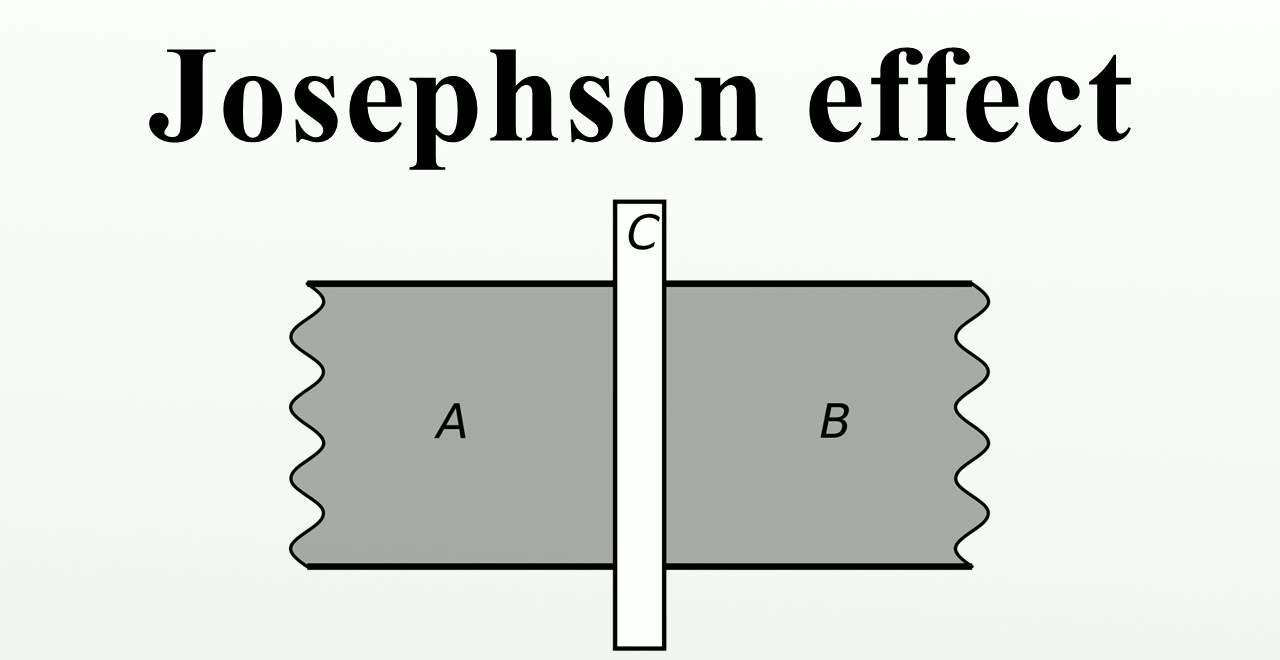
The Josephson effect is a fascinating phenomenon in physics that has had a significant impact on quantum mechanics and superconductivity. Named after Brian David Josephson, the British physicist who predicted it in 1962, this effect provides a window into the quantum nature of electrons and their behavior in certain materials.
In this article, we will delve into 19 astounding facts about the Josephson effect, shedding light on its importance, applications, and implications for our understanding of the quantum world. Whether you are a physics enthusiast, a student studying quantum mechanics, or simply someone curious about the strange and intriguing realms of science, prepare to be amazed by the wonders of the Josephson effect.
Key Takeaways:
- The Josephson effect, discovered by physicist Brian David Josephson, revolutionized superconductivity and led to innovations in high-speed electronics, quantum computing, and medical diagnostics.
- This phenomenon, based on quantum tunneling, enables zero-resistance current flow, precise voltage standards, and the quantization of magnetic flux, paving the way for energy-efficient computing and fundamental physics studies.
Nobel Prize
Brian David Josephson was awarded the Nobel Prize in Physics in 1973 for his discovery of the Josephson effect. His groundbreaking work revolutionized the field of superconductivity.
Quantum Tunneling
The Josephson effect is a manifestation of quantum tunneling, a phenomenon where particles can pass through potential barriers that would be classically impassable. In this case, it refers to the tunneling of Cooper pairs of electrons across the insulating barrier.
Superconducting Junctions
The Josephson junction, which consists of two superconducting materials separated by an insulating barrier, is the fundamental building block of devices that utilize the Josephson effect.
Zero Resistance
When a voltage difference is applied across a Josephson junction, the resulting current flows without any resistance. This property has important implications for the development of high-speed electronic devices.
Frequency Standards
The Josephson effect is used in the creation of highly accurate frequency standards. The frequency of the alternating current generated by the Josephson effect is directly proportional to the applied voltage, making it an excellent reference for precision measurements.
Quantum Voltage Standard
The Josephson effect also provides a way to define the unit of voltage in terms of fundamental physical constants. The Josephson voltage standard offers a precise and reproducible reference for voltage measurements.
Josephson Junction Arrays
Arrays of Josephson junctions can be used to create superconducting digital circuits. These circuits operate at extremely low power levels and have the potential to revolutionize the field of digital electronics.
Superconducting Quantum Interference Devices (SQUIDs)
The Josephson effect is used in the creation of highly sensitive magnetic field detectors called SQUIDs. These devices are widely used in scientific research and medical diagnostics.
Magnetic Resonance Imaging (MRI)
SQUIDs based on the Josephson effect are essential components in modern MRI machines. They allow for the precise detection of magnetic signals from the human body, aiding in the diagnosis of various medical conditions.
Quantum Computing
The Josephson effect is a promising candidate for building qubits, the basic units of information in quantum computers. Its ability to generate and manipulate quantum states makes it an important tool in the development of quantum computing technologies.
Josephson Plasma Oscillations
The Josephson effect can lead to the generation of plasma oscillations in superconducting materials. These oscillations have unique properties and can be harnessed for various applications in physics and technology.
Josephson Voltage Steps
Under certain conditions, the Josephson effect can produce quantized steps in the voltage across a Josephson junction. These steps are a consequence of the discrete nature of electrical charge and can be used for precise measurements.
Quantum Interference Devices
The Josephson effect relies on quantum interference phenomena to produce its unique electrical properties. The coherent flow of Cooper pairs through the junction creates interference patterns that can be controlled and manipulated.
Flux Quantization
The Josephson effect allows for the quantization of magnetic flux. The flux through a superconducting loop containing Josephson junctions is quantized, meaning it can only take on discrete values.
Rapid Swapping
The Josephson effect enables rapid swapping of electrical current between different superconducting pathways. This switching capability has practical applications in the design of electronic circuits and superconducting devices.
Quantum Phase Slips
In certain conditions, the Josephson effect can result in the occurrence of quantum phase slips. These slips are analogous to the spontaneous breaking of electrical symmetry and can lead to novel physical phenomena.
High-Speed Electronics
Superconducting devices based on the Josephson effect have the potential to revolutionize high-speed electronics. Their ability to switch currents without resistance allows for the development of ultrafast and energy-efficient electronic systems.
Energy-Efficient Computing
Quantum computing architectures utilizing the Josephson effect have the potential to revolutionize the field of energy-efficient computing. These systems can perform complex computations while consuming significantly less energy than classical computers.
Fundamental Physics Studies
The Josephson effect has provided physicists with a powerful tool for the study of fundamental physical phenomena. It has enabled detailed investigations into quantum tunneling, quantum coherence, and other quantum effects.
In conclusion, the Josephson effect is a remarkable discovery that has had a profound impact on various fields of physics and technology. Its practical applications in areas such as superconducting electronics and quantum computing continue to push the boundaries of scientific progress.
Conclusion
The Josephson Effect is a truly fascinating phenomenon in the field of Physics. With its ability to create pairs of electrons that behave as a single entity, it has opened up new possibilities for quantum computing and other advanced technologies. This effect, discovered by Brian David Josephson, has not only deepened our understanding of superconductivity but also paved the way for groundbreaking research in the field of condensed matter physics.
By harnessing the Josephson Effect, scientists have been able to design and create highly sensitive sensors, ultrafast digital-to-analog converters, and even quantum voltage standards. The impact of this discovery is immense and continues to shape the future of technological advancements.
As we continue to delve deeper into the mysteries of the Josephson Effect, we can expect even more astounding breakthroughs and applications that will revolutionize various industries and push the boundaries of what we once thought was possible.
FAQs
1. What is the Josephson Effect?
The Josephson Effect is a phenomenon in which pairs of electrons, known as Cooper pairs, tunnel through a barrier between two superconducting materials without any resistance. This effect was discovered by Brian David Josephson in 1962.
2. How does the Josephson Effect work?
The Josephson Effect occurs due to quantum mechanical effects. When a voltage is applied across two superconductors separated by a thin insulating barrier, Cooper pairs tunnel through the barrier, resulting in a flow of supercurrent without any dissipation of energy.
3. What are some applications of the Josephson Effect?
The Josephson Effect has numerous applications, including in superconducting quantum interference devices (SQUIDs) for measuring extremely weak magnetic fields, ultra-sensitive sensors, quantum computing, and high-precision measurements of voltage and current.
4. Can the Josephson Effect be observed in everyday life?
While the Josephson Effect itself may not be directly observable in everyday life, its applications have had a significant impact. For example, superconducting quantum interference devices (SQUIDs) based on the Josephson Effect are used in medical diagnostics, such as measuring brain activity and detecting abnormalities in the human body.
5. Are there any ongoing research efforts related to the Josephson Effect?
Yes, research in the field of the Josephson Effect is ongoing. Scientists are continually exploring new materials and new ways to harness this effect for various applications, such as improving the performance of superconducting qubits for quantum computing and developing more efficient sensors.
Intrigued by the mind-boggling implications of the Josephson Effect? Keep exploring this fascinating phenomenon with our article on 18 astonishing facts that further illuminate its significance. From groundbreaking applications in quantum computing to its role in unlocking the secrets of superconductivity, there's still much more to uncover about this incredible discovery. Join us as we continue to delve into the awe-inspiring world of the Josephson Effect and its profound impact on modern physics and technology. Don't miss out on the chance to expand your knowledge and be amazed by the limitless potential of this remarkable phenomenon!
Was this page helpful?
Our commitment to delivering trustworthy and engaging content is at the heart of what we do. Each fact on our site is contributed by real users like you, bringing a wealth of diverse insights and information. To ensure the highest standards of accuracy and reliability, our dedicated editors meticulously review each submission. This process guarantees that the facts we share are not only fascinating but also credible. Trust in our commitment to quality and authenticity as you explore and learn with us.